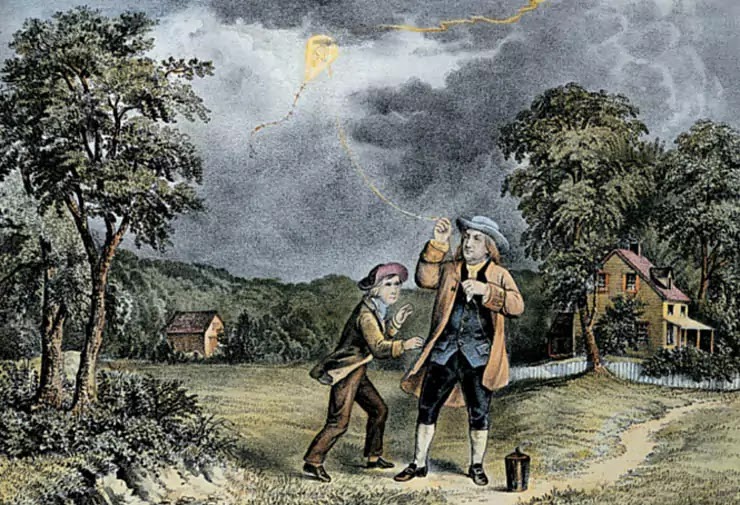
4. The Flying Boy
London, 1730 AD
When the Fellows of the Royal Society of London were invited, on this spring evening, to a “special demonstration,” none of them knew exactly what to expect. That in itself wasn’t saying much. After all, for this group, who fancied themselves the most cutting-edge scientific club in the world, the unexpected was always expected. But today the circumstances were stranger than usual.
The setting was strange, for starters. The Charter House, a sprawling mansion at the northern edge of the city, was a former monastery from which the monks had been kicked out and brutally executed. It had since been converted into a combination of a hospital, a school, and a charity home for “gentlemen” who had fallen on hard times. And one of those charity cases was the man leading the demonstration.
If most of his audience this evening were wealthy, polished, and highly educated at the finest schools in England, Stephen Gray was just the opposite. A stooped, shabbily dressed, painfully shy old man, he had come from humble beginnings and would soon come to a humble end. Born into a family of carpenters and dyers, he had quickly discovered that he was the odd one out, for he had a burning passion for science. Reading everything he could get his hands on, he had taught himself what he needed to know to begin his own experiments.
In the beginning, they were simple, clumsy experiments, carried out with tools that he cobbled together himself. As time went on, he managed to build an impressive amateur laboratory, putting all of the money he earned and all of the free time he could find toward his beloved science. He turned his attention to everything from sunspots to magnets to fossils, and even spent time investigating the “Canterbury ghost,” the most famous apparition of his day. When his bad back made it too difficult to hold down a job, he applied to the Charter House for help and was accepted. In his new home, he picked right up where he left off, gaining quite the reputation among his fellow “brothers” for his bizarre machines and startling demonstrations. And lately, this unlikely genius had taken an interest in electricity.
As the Fellows took their seats, Gray quietly began to set up his apparatus. On one side of the makeshift stage, he brought out a device that would have been familiar to all those present. It was little more than a long glass tube on a wooden stand with a hand-cranked wheel attached, but it had become one of the favorite playthings of eighteenth-century scientists. Invented some fifty years earlier, it worked on the same principle as did Thales’ ancient experiments. The glass took the place of the amber; rubbing it with one hand, while spinning it rapidly with the crank, built up enough of the mysterious force to produce sparks and other curious effects. Even now, that force was still barely understood at all. But now, at least, it had a name: electricity, in honor of elektron or amber, the first substance known to possess it. Gray’s device, in other words, was a variation on the world’s first machine built to produce electricity.
The other items on stage seemed oddly random. Over Gray’s head stood a large wooden frame, to which he had tied four silk cords. On the floor he placed three small platforms, and scattered some tiny objects on each. Next he brought out a large roll of string, an oversized book, a stick, and an ivory ball. Finally, disappearing offstage for a moment, he returned with a boy of about eight or nine. A murmur went through the audience, as the Fellows tried to predict what Gray had in store.
He started simply, with a few tricks that most in the audience had probably seen. The first step was to suck the air out of the tube with a hand-pump, a trick that had been found by earlier researchers to enhance certain electrical effects. After a few minutes of winding up his machine, the spinning tube began to crackle faintly with tiny sparks against his hand. Gray dropped a handful of straw next to it and watched the bits fly to the glass and stick there, just as Thales’ straw had stuck to his amber. Then he gave a signal for the lights to be dimmed, and the audience gasped (in spite of themselves) at the sight of a purplish glow inside the tube. Flickering like St. Elmo’s fire, the light from the charged tube was enough to read by – as Gray promptly demonstrated, by turning the pages of his oversized book.
With the lights back on and the crowd warmed up, he turned next to something new. Producing a feather from his pocket, he let it go; the feather floated to the glass, and then, seemingly repelled, floated back towards him. By spinning the tube faster, then slower, he made the feather hover in midair and “dance.” Then, starting fresh with a new feather, he dropped it next to the cork that was stopping up one end of the tube. The feather was pulled to the cork, just as the previous one had been drawn to the glass.
Now here was something, subtle as it seemed, that made the Fellows lean forward in their seats. Gray glanced up to watch the reaction. Until now, everyone had assumed that electricity – whatever it was – could only be “carried” by the thing that had originally been charged. Under Gray’s guidance, though, the charged glass had seemed to pass its attractive force to the cork that was touching it. But was it only some kind of illusion?
Next Gray inserted his long stick into the cork, and attached the ivory ball to the opposite end of the stick. Charging the tube back up, he held the feather next to the ball. Same effect – even though the ball was separated from the glass by a good three feet! Were there any limits to how far the electricity could travel – or what it could travel through? Gray unrolled the string and stretched it from one end of the room to the other, hanging it from the silk cords. With one end of the string inserted into the cork, and the other end tied by Gray’s assistant to the ivory ball, the electricity seemed to travel – and travel instantly – across the entire distance between the two men. Another gasp went up from the audience as the feather danced next to the ivory, seized just as powerfully as it had been by the tube itself.
But as impressive as these demonstrations had been, Gray was saving his real show-stopper for last. The Society watched with amusement as he carefully lifted the boy into the air, with his assistant’s help, and tied the silk cords to his arms and legs. When he let go, the boy was dangling helplessly in midair, face-down, limbs splayed out like a frog. Again, Gray charged his tube.
What would happen next? Clearly, the wizard of Charter House was building up to something. He had proven that the electric force could be passed from one object to another, and that it could be passed between very different types of objects, and across surprising distances. Did he now mean to send it through the human body itself? Could that be possible? Could it be safe?
Gently, Gray nudged the boy’s foot towards the charged glass, bringing the two into contact. Manning the wheel, his assistant cranked madly. If anything awful was happening to the child, it certainly couldn’t be seen, or heard. After a minute of this, Gray motioned toward the three platforms where bits of paper, straw, and soot were scattered. The boy stretched his hands out towards them – and sure enough, the objects rose into the air like magic to meet him. A round of applause burst from the crowd.
On a roll now, Gray had the boy hover over the book, turning its pages without touching them, wielding only his invisible threads of electric energy. Then he asked for a volunteer to come forward, and had him step onto a platform. The volunteer held hands with the boy, feeling nothing – and then found himself to have inherited the charge, summoning the straw with his newly powerful fingers. A second volunteer was called up, only this one was not given a platform to stand on. He too reached out for the boy, eager to be charged like his colleague – only to recoil in literal shock, as a sharp crack and a visible spark showed that he’d gotten more than he’d bargained for!
Stephen Gray’s show caused a sensation. Soon it was being staged, with tweaks and embellishments, in salons all over the continent. And besides making for great theater, his experiments were nothing less than revolutionary for human knowledge. His modest observation about the charged cork, and all of the more dramatic findings that stemmed from that, opened the door to a new science of electricity. What had been a mind-bending curiosity now became the focus of great practical interest, as experimenters and inventors realized that the electric force could be harnessed and sent over great distances. (Stretching ropes around his friend’s country estate, in a crude version of what would much later be called a telegraph cable, Gray himself managed to pass a charge along over a distance of eight hundred feet!)
Gray’s career, unlikely enough during his lifetime, would not have been possible in an earlier age. But thanks to the spread of printed books, more people had access to knowledge than ever before. As experimenters found instruments and methods that worked, they published their findings, making sure that others wouldn’t repeat their mistakes. Scientific clubs like the Royal Society, although they could be snooty and exclusive, helped turn science from isolated guesswork into an organized, collaborative practice. And kings and parliaments, recognizing that scientific progress was key to staying ahead in the technological race, got into the act by sponsoring this work.
Yet for all its advances, electrical science was still just a baby – “the youngest daughter of the sciences.” It had a long way to go to catch up with a field like astronomy, where superstition and guesswork had been pushed aside by men like Copernicus (who proved that the Earth was not the center of the universe) and Newton (who proved that the planets circled the Sun through the action of a universal force called gravity). Gray and his colleagues still fumbled for the right terms to explain what they were seeing. When an object was charged by another object, what was really happening? Were a stream of invisible particles – “electrical effluvia,” in Gray’s own words – whizzing through the air? When the objects attracted one another, were the particles trying to get back to their source? But then why did charged objects sometimes repeal each other instead?
Certain objects could carry, or “conduct,” electricity better than other objects – that much was clear. Electricity and light were somehow connected, as anyone who’d seen Gray’s glowing tube would agree. And electricity was fast and powerful – although no one could have imagined just how fast and powerful it would turn out to be. The next generation of “electricians” would push the science in thrilling new directions, and they would have Stephen Gray to thank.
The rest of his story is both happy and sad. Shortly after his amazing demonstration, the Royal Society – putting aside their snobbishness – voted to make Gray their newest Fellow. They even gave him a medal, the Copley Medal, making him the very first recipient of what is still one of the most sought-after prizes in science. When he died at Charter House, not six years later, he was still as poor as ever. Yet he was thinking and experimenting until the last, as the Society’s secretary found when he visited Gray on his deathbed. “If God would spare his Life but a little longer,” the secretary reported, “he hoped…to be able to astonish the World with a new Sort of Planetarium…”
5. Lightning in a Bottle
Philadelphia, 1752 AD
It was a muggy afternoon in June, and the sky was growing restless. As the sun vanished behind a swelling gray cloud, and thunder grumbled in the distance, the people of Philadelphia gathered their things and headed indoors. A few of them, on their way home, glanced up to note an unexpected sight: the figures of two men, headed not toward shelter but straight out into the fields surrounding the city. One of them seemed to be carrying a child’s kite.
Had they lost their minds? One or two of the passersby thought of dashing over and warning the loonies about the coming storm. But then they recognized the familiar profile of Mr. Franklin, and turned away with a chuckle. Whatever he’s up to, they thought, I’m sure he has his own, peculiar reasons.
At the age of 46, Benjamin Franklin was the best-known personality in Philadelphia. He had founded the city’s leading newspaper, its volunteer fire department, and its first college, and was currently representing it in the state legislature. Under the name “Poor Richard,” he published a wildly popular “Almanack” each year that served as a grab bag of poems, witty one-liners, brain-teasers, and weather forecasts. In his free time he mastered the violin, guitar, and harp, and developed himself into one of the best chess players in America. Not content with all of that, he had started pouring his energies into science, founding a club called the American Philosophical Society (fashioned in part after the Royal Society of London) in order to have an outlet for his constant flow of ideas.
Not unlike Stephen Gray, Franklin had come from an unlikely background. (In fact, his father had even worked as a dyer like Gray’s, before changing his trade to candle-making.) Not unlike Gray, he had felt out of place for as long as he could remember: one of seventeen children, he had run away as a teenager in search of new challenges. Not unlike Gray, he had a wildly restless, wide-ranging mind, one that seemingly never met a subject that didn’t spark intense curiosity. And very much like Gray, he had been obsessed with electricity from the moment he learned of it.
Unlike Gray, of course, Franklin was an American. And one different thing about America was that it had more thunderstorms, and much more lightning. So perhaps it was not surprising that he fixated on lightning as the phenomenon on which he would build his name as a scientist.
Lightning was certainly not unknown in Europe, and the similarities between it and the electricity that could be produced with amber or glass were obvious. Several people had suggested over the past century that the two forces might be one and the same. In a personal notebook from 1749, Franklin listed out some of the evidence:
Electrical fluid agrees with lightning in these particulars: 1. Giving light. 2. Color of the light. 3. Crooked direction. 4. Swift motion. 5. Being conducted by metals. 6. Crack or noise in exploding. 7. Subsisting in water or ice. 8. Rending bodies it passes through. 9. Destroying animals. 10. Melting metals. 11. Firing inflammable substances. 12. Sulphureous smell.
He went on to mention one of the more recent findings about electricity: that it seemed to be attracted to pointed objects, the sharper the better. Does lightning behave the same way? As far as he was aware, nobody knew. Franklin ended the notebook entry with a command to himself: “Let the experiment be made.”
Now, three years later, he had finally figured out how to perform that experiment. In fact, what he had in mind was quite a bit more ambitious than his original idea. Not only would he try to attract the lightning, he would try to collect its “fire,” in hopes of proving that it was the same as electricity from the laboratory. As the first heavy drops fell on that Philadelphia field, where he stood unpacking his supplies, Franklin couldn’t help feeling nervous. It was well known that lightning had more than enough power to kill. Summoning it to Earth – and not only that, but toward one’s body – would strike many people as sheer madness. It was partly for that reason that he had told no one about today’s experiment, aside from his son, who would serve as his assistant. He glanced up at the dark sky and said a silent prayer.
To speak of “collecting” electricity would have been nonsense in the days of Stephen Gray. Back then, if you wanted to do anything at all with electricity, you had to crank up your generator and use what it produced on the spot. No sooner had a burst of energy come off of that glass tube than it seemed to want to dissipate itself through the quickest route possible. But just a few years after Gray’s death, a new device had been invented that changed all that – and sent shock waves, so to speak, through the scientific community.
As with so many great inventions, the Leyden jar had been discovered more or less by accident. A Dutch scientist, Pieter van Musschenbroek of Leyden, was fooling around in his lab with a glass generator, some wire, and a jar of water, looking for a way to reliably produce big sparks. If he dipped the end of the wire into the water and ran the generator so as to “fill” them both with charge, and then touched the wire with a finger, a fairly impressive spark would result. This was hardly a major breakthrough. But one day a friend of Musschenbroek’s, a lawyer with a casual interest in electricity, happened to stop by. Impressed with his friend’s latest trick, he decided to try to reproduce it at home. It was a decision he would regret.
The lawyer, whose name was Andreas Cunaeus, forgot one crucial detail of Musschenbroek’s setup. His jar of water had been resting on a stand, which was made of an insulating material – that is, one that would not conduct electricity (so that, Musschenbroek reasoned, the charge in the water wouldn’t leak away through the jar). Without anything handy to set the jar on, Cunaeus just held it in his hand. After charging the jar, he reached a finger towards the wire, expecting a mild jolt like he’d felt in his friend’s lab. Instead he got a shock that almost knocked him senseless.
When Cunaeus had regained his wits, he ran straightaway to Musschenbroek to tell him about the awful power he had somehow unleashed. Curious and a bit skeptical, the scientist asked his friend for all the details of his accidental “experiment,” and then started setting things up to try it himself. But your generator is stronger than mine, warned Cunaeus – you don’t know what you’re in for! Musschenbroek laughed and waved a hand at his friend, saying: How bad could it be? With the jar charged to his satisfaction, he stuck out a finger.
Two days later, he was still recovering. He wrote to a scientist friend: “I would like to tell you about a new but terrible experiment, which I advise you never to try yourself, nor would I, who have experienced it and survived by the grace of God, do it again for all the kingdom of France.” The shock had hit him with such force that his whole body had been left shaking like a leaf in a windstorm. “The arm and the entire body are affected so terribly I can’t describe it. I thought I was done for.” At the end of his letter, he confessed that everything he thought he knew about electricity had been turned upside down: after his near-death experience, he now “understood nothing and could explain nothing.”
Electricians around the world, including Benjamin Franklin, rushed to figure out just what Musschenbroek had stumbled upon. Everyone had to experience the effect for himself, of course – which led to reports of fevers, convulsions, nosebleeds, and worse. (One scientist tried the jar on his wife, and left her temporarily paralyzed.) Some began to worry that their line of work was becoming too dangerous. “Would it not be a fatal surprize,” one speculated, “to the first experimenter who found a way to intensify electricity to an artificial lightning?”
For Franklin it was a chance to try out a new theory he’d been working on. For some time he’d had a hunch that electricity was less like a stream of particles and more like a fluid, able to fill any substance. The total amount of this fluid must always stay the same, he thought, so whenever an object got more of it, the object “giving” the fluid must be left with an equal amount less.
Trying to think these new ideas through, Franklin began to feel “a Want of Terms,” as he wrote to a friend. So he set about inventing his own. Objects with extra charge he started to call positive, and those which had given up charge he called negative. Negatively charged objects, he decided, always wanted to get back to their original state – so they acted like sponges, sucking charge from their positive neighbors. Meanwhile, when two positively charged objects got near each other, the excess of charge formed a sort of invisible bubble around each – which explained why they seemed to push against each other.
Franklin’s theory wasn’t perfect, but his new language proved very useful for explaining the “terrible” new Leyden jar. The operation of the generator caused charge to build up in the water, making it positive. (Since his time, thanks to a misunderstanding, his terms have gotten switched – so that we now think of electric charge, for no good reason, as “negative.”) But according to Franklin’s theory, the total amount of charge must remain the same. So where was the negative charge that offset the new positive charge? According to him, it was in the hand holding the jar. Although electricity couldn’t pass through the glass of the jar itself, the two sides somehow “communicated” with each other, the change on the inside inducing the exact opposite change on the outside. When the circuit was connected by touching the wire, the extra charge would eagerly rush into the finger like water through an opened floodgate.
The next few years were devoted to, first, making sure the Leyden jar was safe enough to use, and second, figuring out just how powerful it could be. One scientist determined that instead of continuing to sacrifice human bodies to the cause, he could line the inside and the outside of the jar with tinfoil. These could then serve as the positive and negative bodies, and connecting a wire between them would allow the jar to discharge safely. Another discovered that multiple jars could be connected to each other to strengthen the shock even more. (The setup reminded Franklin of a battery of cannons, and this is where we get our term battery, for a device that stores electricity.) And others found that once a jar was charged, it could be left as it was indefinitely, and would still produce just as big of a shock.
Franklin’s own trials with the Leyden jar had their ups and downs. Hoping to entertain his friends in 1750, he invited them over to watch him electrocute a Christmas turkey. But he got carried away with his performance and accidentally sent the charge into himself instead of the bird. The “universal Blow thro’out my whole Body” that followed taught the overeager experimenter a lesson he wouldn’t forget. Feeling sheepish, he wrote to a friend to tell the story, but begged him not to “make it mor Publick, for I am Ashamed to have been Guilty of so Notorious a Blunder.”
With more practice, and with a few upgrades to his equipment, Franklin grew more confident with the safe use of the tool. And so it was that among the items in his suitcase, on that stormy afternoon in Philadelphia, was a Leyden jar of the new and improved, foil-lined design. This is where he hoped to bottle his lightning. Here was his plan.
The clouds would play the role of the generator. The wet kite string would play the role of the conducting wire. (Before he hit on the kite idea, Franklin had thought of climbing to a great height and setting up a pointed metal rod to attract the electricity. The steeple of the city’s new church, just now under construction, would have served perfectly. But he got tired of waiting for it to be finished.) Of course, Franklin knew that if he held the string directly as it was electrified, the current would rush straight into his body, with possibly fatal results. So he took the string at one end and tied on a ribbon of silk, which had been known as an insulating material since Stephen Gray’s time. Where the string met the silk, he tied on his metal house key, and put the tip of the key into the water of the Leyden jar. When the kite picked up a spark, he reasoned, it would fly down the string, ignore the silk and his hand on the other end, and instead flow through the key into the jar.
Was it foolproof? No. And in fact, Franklin began to have his doubts, as a crackling cloud passed right over his kite without seeming to produce any effect at all. He and his son shared a look. By now they were getting unpleasantly wet, not to mention increasing their odds of being electrocuted with every passing moment. Just a few more minutes, thought Ben, and then we’ll pack up and call it a day.
A minute passed, and then two. Franklin sighed. And just then, glancing at the kite string, he noticed something. A few tiny loose threads were sticking straight up from the string, like hair under a staticky comb. With a lump in his throat, he carefully reached a knuckle towards the key.
The shock he felt, small as it was, made him feel like breaking out into a jig. There was the proof he’d been searching for. The great fiery bolts in the sky, and the humble sparks from the electrician’s machine, were one and the same. Lightning was electric.
By the end of the afternoon, Franklin had collected plenty of charge in his Leyden jar. His discovery had huge implications for our understanding of nature. Electricity was not just a weird, occasional thing that happened under special laboratory conditions. It was present everywhere, bathing us in a silent sea of energy. The vast majority of the time, thanks to the delicate balance of positive and negative charges, we were totally unaware of it. But when a disturbance unleashed its potential, its true character was revealed – that of one of the most powerful forces in the known universe.
On a more practical level, Franklin’s ideas promised to help with one of the biggest safety issues of the time. Besides being an annoyance for picnics, Philadelphia’s thunderstorms posed a major threat to its buildings. Because they were made largely of wood and packed tightly together, a fire started by a lightning strike tended to spread quickly, with often devastating consequences. Franklin saw a solution, in the form of the metal rod he’d hoped to use for his experiment: “Would not these pointed Rods probably draw the Electrical Fire silently out of a Cloud before it came nigh enough to strike, and thereby secure us from that most sudden and terrible Mischief!”
In typical fashion, he used himself as the guinea pig. Mounting a nine-foot “lightning rod” on his roof, he ran a wire from it down through the stairwell to his bedroom door. Borrowing a clever idea he’d read about, he split the wire in two and attached a bell to each end. In the six-inch space between the bells he hung a little brass ball. When the wire was charged, the ball would vibrate back and forth in the space, trying to complete the circuit – and ringing the bells in the process. It was not only a safe way to redirect lightning’s energy from the wood of the house, but also a homemade lightning alarm.
Not only did Franklin’s ingenious contraption work, it sometimes produced spectacular effects. One stormy night he was awakened by flashes of light: a stream of electricity, as big around as his finger, was rushing between the two bells and lighting up the whole stairwell like a lamp. (His wife was not quite such a fan of the lights and noises. Once when he was away in London on business, she wrote to him to find out how to disconnect the blasted thing.)
Before long, Ben Franklin’s lightning rods were being installed on church steeples and other tall buildings everywhere. As for the kite experiment, it encouraged a host of copycats, who were not always as careful. A scientist in Russia, trying to summon lightning the following summer, was found dead after neighbors heard a sound like a cannon going off. He had burn marks on his clothes, splits in his shoes, and an awful red blotch where the charge had leapt from the string and into his forehead. The daredevil of Philadelphia – the larger-than-life man who “snatched lightning from the sky,” as a popular saying went – had been lucky.
6. Raising the Dead
London, 1803 AD
On the morning of the eighteenth of January, 1803, at precisely three minutes after eight, George Forster, convicted murderer, slowly climbed the steps to the gallows. After he prayed briefly with Newgate Prison’s chaplain, a cap was placed over his head, and a noose around his neck. The trapdoor beneath his feet gave way, and it was obvious within a few moments that he was dead. Just to make sure, though, the handful of his friends who were gathered around the platform gave his legs a good yank.
This wasn’t to torture him further, but just the opposite: to save him from further indignity. Since the passage of the Murder Act some fifty years earlier, the bodies of all executed murderers were ordered to be sent off to surgeons for dissection. In order to claim their corpses, the surgeons themselves sometimes had to physically tussle with the friends and family of the deceased, who were terrified that the hanged person would be chopped to pieces before he was fully dead. Had they known what was in store for Forster, they might have been even more afraid than usual.
Forster’s limp body was cut down from the rope and put on a cart, to be carried to a nearby house straightaway. Time was of the essence, because this was to be no ordinary dissection. Giovanni Aldini, the Italian scientist who was waiting with his scalpel, needed a corpse that was as fresh as possible – for he was hoping to capture the mysterious force of life itself.
A crowd quickly gathered around the cart and followed it to the house, where Aldini and several surgeons and officials were gathered around an operating table. A strange device, looking like little more than a stack of metal discs, stood next to the table, with wires emerging from its top and bottom. At the end of each wire was a metal rod, which Aldini held in his hand. No sooner was the body in place than the scientist began, pointing to places where he wanted cuts to be made, and then probing into the cuts with his rods.
Although the experts present were prepared for what was about to happen, many in the crowd just outside were not. There were gasps as George Forster’s dead face, prodded by Aldini, began to tremble and grimace. Screams rang out as one of the corpse’s eyes snapped open. Was he coming back to life?
Aldini left the face and went to work on the body. With blood spurting everywhere, Forster seemed to raise his right arm and clench his fist. Aldini ventured in deeper, and got the most impressive effect yet: the dead man’s legs started kicking, for all the world as though he was about to spring from the table and run off.
None of those who witnessed the demonstration would ever forget it. As the story goes, one of the officials in the room died later that day from the sheer horror. It seemed clear that the new generation of mad scientists had discovered the secret of life – and that before long, they would have the terrible power to bring back the dead. Haunted by these events, five-year-old Mary Godwin (later to become Mary Shelley) would later take up her pen and create a figure very much like Aldini. Although the story of Dr. Frankenstein now strikes us as sheer fantasy, for those who first read it, it seemed frighteningly real.
The experiments of Ben Franklin and others had driven home the fact that electricity could kill. But at around the same time, scientists had become very interested in the opposite notion. What if the mysterious force had the ability to stimulate life? What if – and this was a revolutionary idea indeed – electricity was at the very heart of life?
The concept of healing with electricity, of course, dated back to the Romans’ gout-curing torpedo. Possibly it dated much farther back: in parts of Africa, sick babies were traditionally put into tubs with live electric catfishes. But for those who didn’t have easy access to electric fish, the friction machine and the Leyden jar offered revolutionary new pieces of medical equipment. One of the earliest English believers was John Wesley, the famous traveling minister who founded the Methodist movement. In the mid-1700s Wesley often tended the sick around London, working without a license and with patients who couldn’t afford “real” doctors. He claimed to have used shocks from a Leyden jar to cure a young woman who suffered from epileptic seizures, and later began using the treatment for everything from toothaches to ringworms to blindness (along with more mysterious ailments like “Surfeit,” “Gravel,” and “Feet Violently disorder’d”).
Neither Wesley nor anyone else really understood what was going on when electricity relieved certain symptoms. His best suggestion was that the force “separates the clogging Particles of the stagnating Fluids…accelerating likewise the Motion of the Blood.” But whatever the exact mechanism, it was clear to him that electricity was “the nearest an universal medicine, of any yet known in the world.” He was convinced, too, that doctors of the future would appreciate its unlimited potential – unlike doctors of his own time, who looked at Wesley with a combination of suspicion and annoyance.
Meanwhile, every scientist who could get his hands on a live torpedo began tinkering with the creatures, comparing the fish’s shock with that from the Leyden jar. (Some of those that couldn’t “cheated” by electrifying flounders.) When reports started to come out of South America about a supposedly even more powerful fish, the electric eel, that exotic animal quickly became one of the most sought-after pets in Europe. In the summer of 1775, the exciting news spread that an English scientist had connected an eel to a circuit and produced a visible spark. This was the solid evidence that was needed to prove that animal electricity was, in essence, the same thing that the experimenters had been producing in their labs. But when the truly momentous breakthrough came, it involved a different, and much humbler, animal: the frog.
No one was ever shocked by a frog. But for a scientist who suspected that electricity might be found within all living things, the frog offered a very convenient subject. Frogs were easy to find and simple to take apart, and most importantly, they seemed to retain some “spark” of life for an unusually long time – as long as two days – after they died. Experimenters had pumped electric charge into various freshly-dead frog parts and watched them jump or jitter in response. But it seemed to be a huge leap from that simple demonstration to the proof that electricity was naturally present in living organisms. How could you prove such a thing? One man had an idea.
Luigi Galvani was a modest, deeply religious professor of anatomy at the university in Bologna, Italy. He had read with great interest about the various experiments on torpedoes and eels, and had taken note of one finding in particular. When someone had worked up the courage to actually dissect a torpedo, they had found that the electrical organs of the fish were densely covered with nerves. Galvani also knew, from the recent frog-shocking experiments, that nerves seemed to respond more strongly to electricity than any other body tissue. In private he began piecing together a theory: that a kind of “electric fluid” courses through the highly conductive nervous system, and that when it comes into contact with muscles, it causes the body to move.
Galvani’s confidence slowly grew, until by 1780 he was speaking of “that most noble electric fluid on which…life itself seemed to depend.” But he still didn’t have any slam-dunk evidence. So he doubled his efforts, building a laboratory in his house and devoting about half of his time, over the next ten years, to his experiments. At first they seemed to be going nowhere. And then, on January 26, 1781, a most unexpected thing happened.
Galvani had one of his frogs laid out on the table in his usual way, with its top half sliced off and the main nerves exposed where they entered the legs. Across the room, an assistant was winding up a friction machine, getting it ready for the next electrical experiment. The wires that usually connected the frog legs to the generator hadn’t been hooked up yet, and Galvani was absent-mindedly poking at the frog with his scalpel. At that moment, the machine happened to give off a small spark. And at that very same moment, Galvani jumped back in surprise as the frog legs started to violently kick.
For a minute he sat there staring. What had just happened? His mind raced, trying to piece it together. Somehow, through the air between them – and maybe through the metal of the scalpel blade – the machine had sent an electrical signal to the frog. And somehow that signal had triggered the frog’s muscles. The spark had been just a modest one, and nothing else in the room seemed to have been affected in the slightest. Surely it couldn’t have jerked the frog legs all on its own. In any case, nothing that Galvani had ever read could account for this kind of action at a distance. Had he just witnessed the frog’s own electricity in action?
He would later recall that from that moment on, he was “inflamed with an incredible desire and curiosity” – almost as though he had been jolted with electricity himself. For the next five years he worked on reproducing this marvelous accident, tweaking everything he could think of to try to understand how it worked. He took his frogs outside, onto the terrace at the university, to see if they would respond to electricity from the sky. Sure enough, as storm clouds rolled over, the legs began to dance. If nothing else, he decided, he’d invented a very sensitive electricity detector.
In the fall of 1786, the frog man caught another lucky break. (Of course, it was the sort of luck that only an observant, well-prepared experimenter like Galvani could have taken advantage of.) In his usual thorough way, he was trying to see just how sensitive his frog legs were to electricity in the air, by setting them outside during a calm day and watching for action. Sticking brass hooks through the frogs, and hanging them from a nearby iron railing, proved to be the most convenient way to do this. As Galvani was hanging his frogs, he happened to nudge one of them so that a leg touched the railing at the same time the hook did. To his great surprise, the leg gave a kick.
Galvani was inflamed all over again. Where had the electricity come from this time? He brought the frogs inside, where he could be sure there was no influence from the weather, to try and reproduce the effect. In short order he figured out that he could replace the hook and the fence with a simple little wire, with half made of iron and the other half of brass. All he had to do was connect one end to the frog’s nerve, and the other end to the flesh of its leg, and the dead animal would dutifully kick. There it is, he thought: the proof I’ve been searching for. Now there is no doubt that animals themselves are electric. The frog, Galvani suggested, is basically a messier, more complicated Leyden jar.
But when he published his results, not everyone was convinced. One reader who took particular notice was a man named Alessandro Volta, who taught at a rival university just down the road. As much as he respected Galvani, Volta had his doubts about the frogs.
Galvani’s first experiments, showing the action at a distance, could be explained – Volta reasoned – by a phenomenon called the “return stroke.” According to this phenomenon, which had only recently been discovered, a charged object influenced the charge of other objects within its “field of influence.” When the original object was discharged (as happened when Galvani’s machine let off a spark), a current would run through these other objects as well, as the charges within the field tried to balance themselves out again. As for Galvani making his frogs dance with nothing but wires, Volta was suspicious about the fact that two different metals had to be used. How could Galvani be sure that the electricity was coming from the animal itself, and not somehow from the two metals acting together?
Galvani rose to the challenge. Volta’s “return stroke,” he retorted, was a tiny effect, which had never been seen across distances of more than a foot or two; it couldn’t possibly make a frog leap into action from across a room. To the other point, he admitted that he couldn’t fully explain why two metals needed to be involved. But if the electricity came from the metals and not the animal itself, than why did he get a smaller effect if he made the wire longer?
And with that, one of the greatest scientific feuds in history was off and running. The stakes were sky-high. If Galvani was right and life was electric, who knew what might be possible as humanity continued to master electricity? The quickly growing crowd of “galvanists” – including Galvani’s own nephew, Aldini, who would later jolt dead criminals just as his uncle had jolted frogs – became more and more vocal, and more and more frustrated by Volta’s skepticism. Threats were made. As the tension rose, Volta wrote to a friend: “I know those gentlemen want me dead, but I’ll be damned if I’ll oblige them.” Meanwhile Galvani was complaining: “I am attacked by two opposite sects – the scientists and the know-nothings. Both laugh at me, calling me the ‘frog’s dancing master.’ Yet I know that I have discovered one of the greatest forces in nature.”
As it turned out, in a way, both men were right. Although many of Volta’s criticisms of his experiments were spot-on, Galvani’s main insight – that the nervous system worked through electricity to make animals move – was correct. Sadly, he didn’t get to enjoy his vindication. After his beloved wife’s death in 1790, he never again experimented with quite the same gusto. And when Napoleon’s army came across the mountains to conquer Bologna, Galvani’s conscience wouldn’t allow him to swear an oath of allegiance to the new empire. He was promptly banished from his job and all the privileges that came with it, and he died, depressed and penniless, the very next year.
Meanwhile, Volta’s own fortunes were taking a turn for the better. Though he wasn’t thrilled with the new political situation, he worked out an arrangement with Napoleon that let him keep his job. And his observations about Galvani’s experiments opened an unexpected door to one of the greatest electrical discoveries yet.
Volta quickly realized he needed to do some experiments of his own, in order to follow up on his hunch that the difference between the two metals was somehow the key. Hearing about a curious effect that another scientist had stumbled upon, he tried it for himself. The trick was to put two metal pieces of different kinds on either side of your tongue. Lead and silver were the ones used originally, but brass and iron would work, as in Galvani’s wires. When he put one on top of his tongue and the other on the bottom, his mouth was suddenly filled with a sour, acid taste. When he swapped the two, the taste was bitter (alkaline) instead. Something interesting was happening in his tongue, and it seemed to involve no force whatsoever aside from the difference between the metals.
Volta spent some time putting the metals on various parts of his body, and eventually got tired of sacrificing himself. Instead of using a tongue or a nose (or a frog leg), would a simple stand-in work? He soaked a piece of cloth in salty water, hoping it would have some of the same properties as animal tissue, and pressed it between a copper plate and a zinc plate. Then, looking to capture a stripped-down version of “galvanism” in action, he connected a wire from one plate to the other. Sure enough: an electric current began to flow.
So Galvani had been mistaken about this, at least: the mere presence of two different metals in a circuit, along with something to conduct between the two, was enough to produce charge. No frog-magic required. But more importantly, in his quest to prove his rival wrong, Volta had stumbled upon an entirely new way of producing electricity. Because chemistry was only dimly understood at the time, he had no way of explaining it. But once he started improving his initial design, stacking up multiple “sandwiches” of metal and salty cloth, he soon realized how revolutionary this would be. Unlike the friction machine, which had to be laboriously cranked up and carefully controlled – and unlike the Leyden jar, which stored electricity for later use, but could only release it in one great burst – the new “voltaic pile” could be hooked up and stood on the floor, and it would sit there putting out a steady current for a long time.
What Volta had discovered was the first electrochemical cell – a device that today we call a battery. When the news of his discovery broke, in March of 1800, a new century had begun. By the end of that century, electricity would be powering human civilization in unimaginable ways. And they would all depend on getting a steady electrical current to do useful work. Napoleon, for one, had a glimmer of the importance of Volta’s little pile. Bowled over by the professor’s first demonstration in Paris, the soon-to-be Emperor gave his new favorite scientist the title of Count.
In spite of Volta’s great triumph, the work of the galvanists went on. The mechanisms might have been unclear, but no one had disproved the idea that electricity played a crucial part in living creatures. It would take another hundred years before the nervous system started to give up its secrets, and that work would depend in large part on Volta’s newborn field of electrochemistry. In the meantime, experimenters like Aldini adopted the voltaic pile, using it to control animal movements in ways that went far beyond the old dancing-master Galvani.
Their demonstrations were often shockingly gruesome. A German scientist, who worked with decapitated kittens instead of frogs, claimed that he’d replaced their spinal cords with voltaic piles and made them run and jump around the room. And a Scottish professor, according to a newspaper account (which was probably more than a little bit overblown), later outdid even Aldini in the Dr. Frankenstein department. Bringing a freshly hanged criminal into his lecture hall and placing the dead body ghoulishly in an armchair, he pumped current into the corpse from a large voltaic pile. After twitching and jerking for a few minutes, witnesses swore that the dead man actually rose from his seat. As the students around him screamed or fainted, one of the professor’s colleagues leapt into action: snatching a scalpel from the operating table, he stabbed the “creature” in the jugular and watched him collapse for the final time.
Humanity, it seemed, stood on the verge of a God-like power. Whatever the coming century had in store, it surely would look nothing like the last.
7. Fields of Dreams
London, 1813 AD
In an unremarkable working-class neighborhood in the far west of London, an unremarkable young man was getting ready for bed.
The little house that he shared with his mother was not two miles from Newgate Prison, where mad scientists had dared to raise the dead. Two miles as the crow flies, that is. In Michael Faraday’s own mind, an ocean might as well have separated him from sensational events like these. At twenty-one years old, he was only a blacksmith’s son and a bookbinder’s apprentice, with no money and no prospects, let alone fame and fortune. Yet all that was about to change.
Just as he was slipping into his threadbare night-clothes, there was a knock at the door. A visitor at this hour was strange indeed. Michael peeked through the window, and his surprise turned to astonishment. There in the street, as though it had dropped from the sky, was a coach as grand and elegant as something from a fairy tale. Stumbling back into his regular clothes, Michael rushed to the door, gathered himself for a moment, and opened it.
The footman who stood there was every bit as grand and elegant as the coach. He had been sent, he said, by Sir Humphry Davy. Mr. Davy kindly requested the presence of Mr. Faraday the following morning. That was all.
As the coach rolled off, Michael stood there dumbfounded. Anyone in all Britain, and beyond, would have recognized the name of Humphry Davy. In an age when science had never been more glamorous, Davy was the biggest superstar in science. He was brilliant, he was charming, and he was handsome, and it made for a devastating combination. He had served as president of the old Royal Society, among many other accomplishments, but he was best known for his role at the newer Royal Institution. When Davy’s lectures there began to draw crowds by the hundreds, the Institution had been forced to redirect traffic and remodel their building just to keep up with his popularity. Just the previous year, he had been knighted by the King.
Davy’s field was chemistry, where many of the day’s most sensational discoveries were being made – the lion’s share of them, it seemed, by Davy himself. His favorite tool was the brand-new voltaic pile, which he had turned from a plaything into an industrial-strength workhorse, stacking up hundreds and even thousands of metal pieces to drive a powerful current. Davy had been among the first to catch on that the salty stuff between Volta’s discs was helping to produce the current, by splitting apart at its most basic level and reacting with the metal. Now he was using that knowledge to accomplish something that seemed almost magical: separating everyday substances like water and salt into their basic ingredients. In the process he was uncovering what, as far as anyone knew, were the very smallest and most fundamental building blocks of the universe.
Everyone knew of the great Davy. But our young apprentice was not just anyone, and to him Humphry Davy was not just another celebrity; he was a personal hero.
Really, you could only have called Michael Faraday unremarkable if you didn’t know him at all. To those who knew him, it had been clear from the start that he was filled with an extraordinary, unquenchable curiosity. It was a curiosity that couldn’t be kept within the walls of a school; in fact, the child’s one attempt to actually go to school ended almost as soon as it had begun. (His teacher beat him as punishment for not saying his r’s correctly, and Michael’s mother took him out and never looked back.) Instead, he went to work at age thirteen, and lucked into the best of all possible places to do so: the bookshop around the corner.
The eccentric Frenchman who ran the shop took Michael on as an errand boy at first. But when he discovered that he couldn’t keep the boy out of his books, he agreed to begin training him as a bookbinder. Once Faraday’s burning curiosity about nature met up with the world of knowledge in Monsieur Riebau’s science section, the reaction was explosive. A particular favorite was the wondrous Encyclopaedia Britannica, where in volume three, Michael discovered the article titled “Electricity.”
Before long, strange items like homemade Leyden jars were appearing in the bookshop, alongside stacks of notes and sketches. Michael read about the great works of men like Franklin and Galvani, and fantasized about having a laboratory of his own, where he would solve once and for all the mysteries that had tormented men for centuries. In the meantime he made do with the dusty corners of his little house and the shop. And then there was the busy city just outside his door, which, for a mind like his, offered a constant flood of puzzles and inspirations. You can get a peek into that mind by reading his letters to his friends. Here is one:
I set off from you at a run and did not stop until I found myself in the midst of a puddle and a quandary of thoughts respecting the heat generated in animal bodies by exercise. The puddle however gave a turn to the affair and I proceeded from thence deeply immersed in thoughts respecting the resistance of fluids to bodies precipitated in them…My mind was…suddenly called…by a very cordial affectionate and also effectual salute from a spout. This of course gave a new turn to my ideas and from thence to Black Friars Bridge it was busily bothered amongst Projectiles and Parabolas. At the Bridge the wind came in my face and directed my attention…to the inclination of the pavement. Inclined Planes were then all the go and…on the other side of the Bridge…slipping introduced the subject of friction and the best method of lessening it…The Velocity and Momentum of falling bodies next struck not only my mind but my head, my ears, my hands, my back and various other parts of my body…from thence I went home, sky-gazing and earnestly looking out for every Cirrus, Cumulus, Stratus, Cirro-Cumuli, Cirro-Strata and Nimbus that came above the horizon.
Many of the shop’s regular customers, picking up on his eager curiosity, developed a fondness for Riebau’s apprentice. In the spring of 1812, one of them offered Michael a gift that would change his life. He happened to have an extra ticket, he said, for the scientific event of the year: a series of lectures by Humphry Davy himself. These would be no ordinary lectures, because they would serve as Davy’s farewell; the great man had recently married a rich widow, and he would shortly be stepping down from the Royal Institution. Would Michael like to tag along?
Never was there an easier question to answer. And never, even at Davy’s lectures, had there been a more attentive listener. Packed into the Institution’s hall alongside some of the most fashionable figures in London, Michael felt – if this was possible – both completely out of place, and at home like never before. He watched in something like a trance as Davy used his hulking battery to conjure up pure potassium from a paste of salt, then made it dance and fizz in water and finally burst into violet flames. He watched as Davy demonstrated the world’s first electric lamp (an ancestor of today’s fluorescent bulbs), easing two rods of charged charcoal toward each other until a brilliant beam of “lightning” leapt between them. And the whole time he took furious notes.
Back in the dusty quiet of the bookshop, Michael couldn’t get Davy’s voice out of his head. So he went through his notes, cleaning them up, putting them in order, creating as he went a narrative that explained all of the great chemist’s ideas. When he was done, he found that he had what amounted to a three-hundred-page book.
Monsieur Riebau took one look at the enormous thing and sighed. You are not going to be cured of this science business anytime soon, he said. Why not send it to Mr. Davy? Who knows? Maybe he could use a man like you.
To Michael it sounded like the longest of long shots. But what was the alternative? He knew all too well. His apprenticeship was just then coming to an end, and a job had already been lined up for him, working under another bookbinder nearby. His new boss was an old grouch of a man, who surely would have no patience for Michael’s daydreams and contraptions. A life of lonesome drudgery stretched out before him. With nothing to lose, he took a deep breath and mailed the package. To his shock, he got a response.
In Sir Humphry’s own words – words Michael would treasure for the rest of his life – he was “far from displeased” with the contents of the package. But as it turned out, he had no work to offer the young man. Work hard at your bookbinding, he advised, and I’ll be sure to keep you in mind. And that was that. Until now.
Getting dressed in a state of mild panic, the morning after Davy’s fateful coach had arrived at his door, Michael wondered if something had happened to change the man’s mind. He didn’t have to wait long to find out. No sooner had he been ushered into Davy’s office at the Institution than he had, for all intents and purposes, been offered a job. It seemed that one of the chemist’s assistants had accused another of laziness, and the other had promptly answered with his fists. After knocking over some valuable equipment in the course of their brawl, they had been thrown out of the Institution. Needing help quick, Davy decided to take a gamble on the eager youngster Faraday.
Michael’s first months as a professional scientist were not exactly glamorous. He was paid even less than he had been – and to earn even this paltry salary, he had to risk life and limb. He soon found this out the hard way, as he was working with a sample of nitrogen trichloride (an unstable compound that Davy was researching) and accidentally set off an explosion. The blast tore off one of Michael’s fingernails and left scratch marks all over his glass mask, which fortunately he had remembered to wear. He had been lucky; Davy himself had been partially blinded in a similar accident just a few months before.
But all of this mattered little. Young Michael Faraday, scientist in training, had unlimited access to one of the finest laboratories in the world. He had never felt happier or more important. A new challenge was coming, though, that would truly put his enthusiasm to the test.
It started happily enough. Davy announced one day that he was making preparations for a grand tour of Europe. He wanted to meet some of the continent’s great scientists in the flesh, and to study some of its greatest sights firsthand, including the volcanoes of Italy. For Michael, who had never been more than a few miles from London, it sounded like an epic voyage of discovery.
But then Davy revealed that the only way he could bring Michael along was if he took him as a servant. So when they set out that fall, instead of spending all of his time in starry-eyed wonder, Michael found himself spending much of it shaving his master’s whiskers and emptying his chamber pot. While Davy felt guilty about subjecting his brilliant assistant to chores like these, his haughty wife simply ordered Michael around like the peasant she saw in him. Forced to travel second-class and eat with the servants while the Davys enjoyed the finest luxuries of Paris and Rome, he felt yet again the strain between his dreams and his social class. That strain was not lost on the scientists they met during their travels, who expressed wonder to Sir Humphry that his sharp young apprentice could be treated with such little respect.
Michael hated to complain, but in his letters back home he couldn’t help voicing his frustration. Once or twice he even convinced himself he would go back to bookbinding. But the disappointments of the grand tour, in the end, only hardened his determination to make his own name for himself in science.
Back in London, he gradually gained more and more independence, diving into his own experiments instead of simply helping with Davy’s. At first they were tentative and haphazard, although his gifts were obvious. He discovered a few new interesting compounds, invented a form of stainless steel, and spent years trying to develop a better type of glass for use in telescope lenses. (Though the lead-infused “heavy glass” he came up with was basically a failure, it would later open a door to a far greater discovery.) But then a small piece of news made its way to the Royal Institution that would give Michael’s career the structure it lacked – and, in so doing, launch it like a rocket.
The news was of an odd phenomenon that a Danish scientist happened to notice while giving a lecture. As he was running an electric current through a wire, something caught his eye: the needle of a compass that was sitting nearby began to move.
The urge to draw some connection between electricity and magnets was anything but new. Thales himself had pointed out the parallel between amber’s attractive force and that of the magnetic rock called lodestone. True, magnetism couldn’t make sparks, travel through wires, or be passed to different substances, as far as anyone knew. But both of these strange phenomena had that magical quality of action at a distance. And there was evidence for a link, too, from stories of lightning striking houses and turning metal objects inside them into magnets. Surely this couldn’t be pure coincidence.
The year was 1820, and Michael Faraday was now twenty-eight years old. As modest as he was, he couldn’t help feeling the pressure to accomplish something great, something that would raise his name to the starry level of his mentor’s. When he heard about the Danish finding, his intuition lit up like one of Davy’s lamps. Though such a result was easy to dismiss as a simple curiosity, Faraday knew his history well enough to know that it was just this kind of curiosity – a fleeting glimpse of a connection between two different fields of science – that so often “burst open the gates” to great unexplored realms. Gathering a compass, some wire, and a battery, he set about trying to reproduce the effect.
Taking the plunge into electrical research meant diving into some very murky waters. Despite the new gadgets that the past century had come up with, from lightning rods to voltaic piles, science still seemed to be a long way from understanding what electricity was and how it worked. Dr. Franklin’s theory, which claimed that excesses and shortages of “electrical fluid” produced positive and negative charges, had become widely popular – but many still cling to an older theory, which took electricity to be made up of two different fluids called “vitreous” and “resinous.” And neither one of these theories could explain how the same stuff could “flow” through a wire as a current, “leap” through the air as a spark, and then “swim” through the salty goo in a battery (or an animal’s brain).
But if he could work out the mysterious relationship between electricity and magnets, Faraday had a hunch that he could solve these problems and more. The first thing he noticed was that that relationship was not a simple matter of attraction or repulsion. Instead, the compass needle seemed to want to point itself sideways – that is, at a right angle – relative to the flow of the current. He reasoned that the electricity must be creating an invisible force-field around the wire that was circular in shape. Magnets, of course, produce their own invisible fields; you can feel their shape by simply moving two magnets around near another. When Faraday brought his wire together with a magnet, sure enough, he could feel their fields interacting with each other – not by pushing or pulling, but by trying to move around one another in a circle. But as soon as he disconnected his battery, the effect vanished.
What if he set up the wire and the magnet so that they could move freely? The way he accomplished this was both simple and brilliant. He propped up a magnet in a glass filled with conductive liquid (he used mercury, but even salt water would work), so that its tip was barely sticking out above the surface. Then he dangled a wire into the glass, using a cork to make the tip of the wire float on the surface. He put the end of another wire into the liquid, and then completed the circuit with a battery.
The moment he did so, something wonderful began to happen. As the current flowed from the battery through the liquid into the dangling wire, creating a field around itself, the wire began to move. Guided invisibly by the clashing fields, the tip of the wire spun in circles around the magnet’s pole. Around and around it went, happily skating across the surface for as long as the battery would hold out.